Electric current: Definition, symbol, formula, Unit & Types
Electric current is the rate of flow of charges through a cross-sectional area. It is a scalar quantity. SI unit of current is Ampere that is equal to coulomb per second. If you want to learn about current in detail, read this post:
This post includes:
- Definition of electric current
- Electric current formula
- Unit of current
- Types of electric current
- Current density and much more
Keep reading …
Electric current definition
Under certain conditions, in conductors, the continuous ordered motion of free charge carriers can occur. Such a movement is called an electric current. The direction of movement of positive free charges is taken as the direction of the electric current, although in most cases electrons move — negatively charged particles.
Electric Current formula
A quantitative measure of the electric current is the current strength I – a scalar physical quantity equal to the ratio of the charge q transferred through the conductor cross-section during the time interval t to this time interval:

If the current is not constant, then to find the amount of charge passed through the conductor, calculate the area of the figure under the graph of current versus time.
If the current and its directors do not change with time, then this current is called constant. The current is measured by an ammeter, which is included in the circuit in series. In the International System of Units of SI, the current strength is measured in amperes [A]. 1 A = 1 C / s.
The average current is found as the ratio of the total charge to the entire time (i.e., according to the same principle as the average speed or any other average value in physics):

If the current changes uniformly over time from the value of I 1 to the value of I 2 , then the average current value can be found as an arithmetic average of the extreme values:

Current Density:
Current density – current strength per unit of conductor cross-section, is calculated by the formula:

When current passes through a conductor, the current experiences resistance from the conductor side. The reason for the resistance is the interaction of charges with the atoms of the substance of the conductor and with each other. The unit of measurement is 1 Ohm. Conductor resistance R is determined by the formula:

where: l is the length of the conductor, S is the area of its cross-section, ρ is the resistivity of the conductor material (be careful and do not confuse the last value with the density of the substance), which characterizes the ability of the conductor material to resist the passage of current. That is, it is the same characteristic of a substance as many others: specific heat capacity, density, melting point, etc. The unit of measurement of resistivity is 1-ohm · m. The resistivity of a substance is a tabular value.
The resistance of the conductor depends on its temperature:

where: R 0 is the conductor resistance at 0 ° С, t is the temperature expressed in degrees Celsius, α is the temperature coefficient of resistance. It is equal to the relative change in resistance, with increasing temperature by 1 ° C. For metals, it is always greater than zero, for electrolytes, on the contrary, it is always less than zero.
DC link diode
A diode is a non-linear circuit element whose resistance depends on the direction of current flow. Denoted by the diode as follows:

The arrow in the schematic designation of the diode shows in which direction it transmits current. In this case, its resistance is zero, and the diode can be replaced simply with a conductor with zero resistance. If the current flows through the diode in the opposite direction, then the diode has an infinitely large resistance, that is, it does not let the current through at all, and is a break in the circuit. Then the section of the circuit with a diode can be simply crossed out since no current flows through it.
Ohm’s law
The German physicist G. Ohm experimentally established in 1826 that the strength of current I flowing through a uniform metal conductor (that is, a conductor that does not have external forces) with resistance R is proportional to the voltage Uat the ends of the conductor:

The value of R is called electric resistance. A conductor with electrical resistance is called a resistor. This ratio expresses Ohm’s law for a homogeneous section of the circuit: the current in the conductor is directly proportional to the applied voltage and inversely proportional to the resistance of the conductor.
Conductors obeying Ohm’s law are called linear. The graphical dependence of the current I on the voltage U (such graphs are called current-voltage characteristics, abbreviated VAC) is represented by a straight line passing through the origin. It should be noted that there are many materials and devices that do not obey Ohm’s law, for example, a semiconductor diode or a gas discharge lamp. Even with metal conductors at sufficiently high currents, there is a deviation from Ohm’s linear law, since the electrical resistance of metal conductors increases with increasing temperature.
Conductors in electrical circuits can be connected in two ways: in series and in parallel. Each method has its own laws.
1. Patterns of serial connection:

The formula for the total resistance of series-connected resistors is valid for any number of conductors. If the circuit is consistently included n identical resistances R , then the total resistance R 0 is found by the formula:

2. Patterns of parallel connection:

The formula for the total resistance of parallel-connected resistors is valid for any number of conductors. If, in parallel, the circuit includes n identical resistances R, then the total resistance R 0 is found by the formula:

Electrical measuring instruments
To measure voltages and currents in DC electric circuits, special devices are used – voltmeters and ammeters .
A voltmeter is designed to measure the potential difference applied to its terminals. It is connected in parallel to the circuit section where the potential difference is measured. Any voltmeter has a certain internal resistance R B . In order that the voltmeter does not introduce a noticeable redistribution of currents when connected to the circuit to be measured, its internal resistance must be large compared to the resistance of the circuit to which it is connected.
Ammeter is designed to measure the current in the circuit. The ammeter is connected in series to an open circuit so that all measured current passes through it. Ammeter also has some internal resistance R A . Unlike a voltmeter, the internal resistance of an ammeter must be sufficiently small compared to the total resistance of the entire circuit.
Emf. Ohm’s law for complete chain
For the existence of direct current, it is necessary to have a device in an electric closed circuit capable of creating and maintaining potential differences in the circuit sections due to the work of forces of non-electrostatic origin. Such devices are called DC sources . Forces of non-electrostatic origin acting on free charge carriers from current sources are called extraneous forces .
The nature of external forces may be different. In galvanic cells or batteries, they arise as a result of electrochemical processes; in DC generators, external forces arise when conductors move in a magnetic field. Under the action of external forces, electric charges move inside the current source against the forces of the electrostatic field, so that a constant electric current can be maintained in a closed circuit.
When electric charges move along the DC circuit, external forces acting inside the current sources do the work. Physical quantity equal to the ratio of the work A st external forces when moving charge q from the negative pole of the current source to positive to the value of this charge is called the source electromotive force (EMF) :

Thus, the EMF is determined by the work done by outside forces when moving a single positive charge. The electromotive force, as well as the potential difference, is measured in volts (V).
Ohm’s law for a complete (closed) circuit: the current in a closed circuit is equal to the source electromotive force divided by the total (internal + external) resistance of the circuit:

Resistance r is the internal (intrinsic) resistance of the current source (depends on the internal structure of the source). Resistance R – load resistance (external resistance of the circuit).
The voltage drop in the external circuit at the same time (it is also called the voltage at the source terminals ):

It is important to understand and remember: the EMF and the internal resistance of the current source do not change when different loads are connected.
If the load resistance is zero (the source is closed on itself) or much less than the source resistance, then a short-circuit current will flow in the circuit :

Short-circuit current is the maximum current that can be obtained from this source with electromotive force ε and internal resistance r . For sources with a small internal resistance, the short-circuit current can be very large and cause destruction of the electrical circuit or source. For example, in lead batteries used in automobiles, the short circuit current may be several hundred amperes. Especially dangerous are short circuits in lighting networks powered by substations (thousands of amperes). To avoid the destructive effect of such large currents, fuses or special circuit breakers are included in the circuit.
Several sources of emf in the circuit
If there are several emfs connected in series in the circuit , then:
1. With the right (the positive pole of one source joins the negative of the other) connecting sources, the total emf of all sources and their internal resistance can be found by the formulas:

For example, such a connection of sources is carried out in remote controls, cameras and other household appliances that operate on multiple batteries.
2. When the wrong (sources are connected by the same poles) connecting sources, their total emf and resistance is calculated by the formulas:

In both cases, the total resistance of the sources increases.
With a parallel connection, it makes sense to connect sources only with the same EMF, otherwise the sources will be discharged against each other. Thus, the total emf will be the same as the emf of each source, that is, with a parallel connection, we will not get a battery with a large emf. This reduces the internal resistance of the battery sources, which allows you to get more current and power in the circuit:

This is the meaning of the parallel connection of sources. In any case, when solving problems, you first need to find the total emf and total internal resistance of the resulting source, and then write down Ohm’s law for the complete circuit.
Joules law of heating
Operation A of the electric current I flowing through a fixed conductor with resistance R is converted into heat Qreleased on the conductor. This work can be calculated by one of the formulas (taking into account Ohm’s law, they all follow from each other):

The law of transformation of current into heat was experimentally established independently of each other by J. Jole and E. Lenz and is called the Joule – Lenz law. The power of the electric current is equal to the ratio of the work of the current A to the time interval Δ t for which this work was done, so it can be calculated using the following formulas:

The work of electric current in SI, as usual, is expressed in joules (J), power-in watts (W).
Closed Circuit Power Balance
Consider now the full DC loop consisting of a source of electromotive force ε and internal resistance r and the outer portion of uniform resistance R . In this case, the net power or power released in the external circuit:

The maximum possible effective power source is achieved if R = r and is equal to:

If, when connected to the same current source of different resistances R 1 and R 2 , they are allocated equal power, then the internal resistance of this current source can be found by the formula:

Power loss or power inside current source:

The total power developed by the current source:

Current source efficiency:

What is Electrolysis?
Electrolytes are called conductive environments in which the flow of electric current is accompanied by the transfer of matter. The carriers of free charges in electrolytes are positively and negatively charged ions. Electrolytes include many metal compounds with metalloids in the molten state, as well as some solids. However, the main representatives of electrolytes, widely used in engineering, are aqueous solutions of inorganic acids, salts, and bases.
The passage of electric current through the electrolyte is accompanied by the release of a substance on the electrodes. This phenomenon is called electrolysis.
The electric current in electrolytes is the movement of ions of both signs in opposite directions. Positive ions move to the negative electrode ( cathode ), negative ions move to the positive electrode ( anode ). The ions of both signs appear in aqueous solutions of salts, acids, and alkalis as a result of the splitting of a part of neutral molecules. This phenomenon is called electrolytic dissociation.
The law of electrolysis was experimentally established by the English physicist M. Faraday in 1833. The Faraday law determines the number of primary products released on the electrodes during electrolysis. So, the mass m of the substance released on the electrode is directly proportional to the charge Q that passes through the electrolyte:
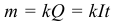
The value of k is called the electrochemical equivalent. It can be calculated by the formula:

where: n is the valence of the substance, N A is the Avogadro constant, M is the molar mass of the substance, and e is the elementary charge. Sometimes the following designation is also introduced for the Faraday constant:

The electric current in gases and in vacuum
The electric current in gases
Under normal conditions, gases do not conduct electrical current. This is due to the electrical neutrality of the gas molecules and, therefore, the absence of carriers of electric charges. In order for a gas to become a conductor, it is necessary to detach one or more electrons from the molecules. Then free charge carriers will appear – electrons and positive ions. This process is called the ionization of gases.
Ionize gas molecules can be an external influence – an ionizer. Ionizers can be a stream of light, X-rays, a stream of electrons or α- particles. Gas molecules also ionize at high temperatures. Ionization leads to the appearance in the gases of free charge carriers – electrons, positive ions, negative ions (an electron united with a neutral molecule).
If an electric field is created in the space occupied by the ionized gas, then the carriers of electric charges will come in an orderly motion — this is how an electric current arises in gases. If the ionizer stops acting, then the gas becomes neutral again, as recombination occurs in it — the formation of neutral atoms by ions and electrons.
Vacuum current
Vacuum is a degree of rarefaction of a gas, at which a collision between its molecules can be neglected and the average free path is assumed to exceed the linear dimensions of the vessel in which the gas is located.
The electric current in a vacuum is called the conductivity of the interelectrode gap in a state of vacuum. At the same time, gas molecules are so small that their ionization processes cannot provide such a number of electrons and ions that are necessary for ionization. The conductivity of the interelectrode gap in a vacuum can be ensured only with the help of charged particles, arising due to emission phenomena on the electrodes.
See Also: Magnetism